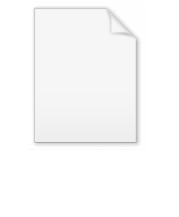
Electroless nickel
Encyclopedia
Electroless nickel is a broad term that incorporates a diverse mix of technology ranging from nickel boron and ternary alloys to highly functional composites. Nickel phosphorus
deposits find the most use in the markets the plating
industry serves.
or wear and in some cases, both. Because of the unique deposit properties and uniformity of the resultant film, many other applications have emerged that continue to capitalize on the chameleon-like nature of electroless nickel. The majority of EN films used commercially are deposited from solutions formulated with sodium hypophosphite as the reducing agent. This results in nickel films that are alloyed with phosphorus in ranges between 1 and 12 weight percent.
The mechanical properties of NiP (ENP) deposits can be further enhanced not only by the co-deposition of inert particles such as teflon, silicon
carbide or boron nitride
but also by alloying with a third element, forming a ternary alloy of NiPX, where X can be copper
, tungsten
, molybdenum
or tin depending on the particular formulation.
Electroless nickel boron (NiB) alloys are also well cited in the literature, although they are less commercially viable than the NiP alloys. The films are generated using either sodium borohydride or dimethylaminoborane as the reducing agent and can range in boron content from 1–5 weight percent. NiB films are typically used in the electronics industry where low-resistivity coatings are required and also find use in industrial applications when extreme wear and increased coating hardness is specified.
Electroless nickel is no different than its electroplated counterparts in that it is only as strong as the weakest link in the process cycle. More clearly stated, successful electroless nickel plating
requires both strict adherence to bath operating guidelines and optimal surface preparation. A well formulated electroless nickel process will not overcome improper surface preparation. Conversely, applying the wrong electroless nickel will short-circuit success even when the substrate is wonderfully prepared. The following links are critical:
An inability to recognize the interconnectivity of these three basic items will ultimately lead to failure somewhere along the supply line, hopefully, before the component heads to the field.
Electrodeposited nickel has a purity of greater than 99% and is highly crystalline
. On the other hand, electroless nickel deposits that contain more than 10.5% phosphorus appear to be amorphous
, i.e., lacking crystal structure. EN deposits with less than 7 weight percent phosphorus have a clear microcrystalline structure (2-6 nm grain size) and film properties are distinctly different. Some studies have found that higher phosphorus deposits (above 10.5 weight percent phosphorus) may not be truly amorphous but rather a mixture of microcrystalline and amorphous phases.
Putting aside some of the well known facts about deposit phosphorus vs various properties, i.e hardness or corrosion resistance that we will cover a bit later, deposits with a high degree of amorphous composition are free of grain boundaries, which typically act as sites for intergranular corrosion commonly encountered in crystalline deposits. There are also applications that benefit from crystalline structure as well. Duncan pointed out that in the as deposited condition an electroless nickel coating can contain both crystalline and amorphous phases or, if dictated by formula, may only contain one. Each phase offers distinct benefits and understanding what exists in the deposit you plan to apply is relevant.
are not a factor, therefore sharp edges, deep recesses and blind holes are readily plated to uniform thickness with electroless nickel chemistry. Many applications for electroless nickel exist today because it is often the only way to plate certain components. The difference in deposit uniformity is illustrated in figure 1. Figure 2 is a photo of EN plated bumps on a flip chip, something impossible to accomplish with an electroplated deposit.
The degree of uniformity can vary on edges, threads, small holes or deep recesses where exchange of fresh solution may be difficult. This can also occur under conditions with excessive bath agitation, especially in the presence of heavy metals. This thickness variation may be controlled by optimizing solution dynamics and/or by controlling the concentration of certain additives formulated into the EN plating bath.
Figure 5 depicts the effect phosphorus content has on ferromagnetic properties.
Not all high phosphorus alloys will maintain the same level of thermomagnetic stability. The deposit performance is also dependent on the chemical formulation and the solution dynamics during the plating process. Reducing the onset and the rate of crystallization, minimizing the volume fraction of microcrystallinity and maintaining a homogeneous grain structure at higher bake temperatures are key requirements for optimization of the films thermomagnetic performance.
or zinc alloy
that protect the base material by sacrificing itself.
The nature of the corrosive environment and the deposits resistance to chemical attack are also important criteria when selecting a particular EN coating for optimum performance. High phosphorus coatings (10-12%) are more readily attacked in strongly alkaline media than low phosphorus deposits (1-3%), however they perform significantly better in acidic media. This is illustrated in the following graphs.
Optimizing the performance of EN coatings for maximum corrosion protection requires that the coating be continuous and free of any microporosity, roughness, nodules and irregularities within the microstructure. Since the microstructure of high phosphorus EN films are amorphous they are essentially free of the grain boundaries that could potentially serve as sites for corrosion. The absence of such phase boundaries and the ability of the high phosphorus EN film to form a passive film on its surface, make it an excellent choice for protection on aluminum and steel substrates in highly corrosive, acidic environments.
The phosphorus content alone however is not enough to maximize the coatings corrosion resistance in a given environment. Factors that will affect the corrosion performance of EN films are:
Surface condition plays an important role in determining the required deposit thickness and good field performance. For example, machined substrates alloyed with sulfur and/or lead are particularly vulnerable to a high degree of porosity, since these alloying constituents are catalytic poisons to the deposition process. Substrates with high roughness values require thicker deposits to provide adequate corrosion protection. This is primarily due to the formation of nodules on the roughened during the initial stages of deposition, resulting in microporosity of the ENP film.
Increasing the deposit thickness can minimize this affect. Smooth substrates require a thickness of 25 microns to obtain a film with minimal porosity whereas roughened surfaces can require up to three times the thickness to provide similar corrosion protection.
The use of neutral salt spray, in accordance with the ASTM B 117 specification, for the measurement of corrosion performance is well documented in the literature and is still the most widely accepted method for coating evaluation. The results of neutral salt spray exposure, phosphorus content and deposit thickness are summarized in the table below:
Heat treatment of ENP deposit will significantly increase the microhardness. Figure 9 illustrates the effective temperature range and time required to attain specific values.
In cases where the substrate cannot withstand the temperature requirements to achieve maximum hardness, low phosphorus EN is often recommended. The increase in microhardness for ENP films is attributed to the phase transformations that take place during the heat cycle to form nickel metal and nickel phosphide. Some volume shrinkage and cracking of the film will occur and must be taken into account if the application requires corrosion protection.
The abrasive wear of ENP films are typically measured by applying a mechanical action of an abrasive, rotating wheel on the plated surface and measuring the weight loss (in milligrams) of the coating at intervals of 1000 cycles. This test is referred to as the Taber Wear Index (TWI) and perhaps is the most frequently used method to evaluate the wear characteristics of ENP films. Typical weight loss results for various deposits are illustrated in Figure 10.
The “as plated” low phosphorus deposits tend to withstand abrasive wear better than the high phosphorus coatings, largely attributed to the higher hardness values. However after heat treatment the trends remain the same even though the deposit hardness is similar suggesting that other factors, such as deposit composition, play a role in abrasive wear resistance.
Adhesive wear is defined as the removal of material between mating surfaces and measures the films ability to resist galling, welding or seizing. If the mating surfaces are both electroless nickel, optimum performance is achieved if the surfaces in contact have dissimilar properties, such as phosphorus content or hardness. The adhesive wear resistance of ENP films improves with increasing phosphorus content.
It is important to consider as many variables as possible when evaluating an ENP coating for tribological applications. Factors such as hardness, phosphorus content, the presence of corrosive liqiuids or gases, the temperature, the nature of the mating surface, the degree of lubrication and the characteristics of the substrate are critical to optimum performance.
is well known as possessing a self healing lubricating film. Whereas EN does not exhibit this feature it still offers excellent lubricity
and finds many uses that can capitalize on this property. Figure 11 graphically displays the coefficient of friction vs. non lubricated steel
. Hard chrome does perform better than standard high phosphorus EN (HP EN) but with occlusion of various soft particles you can achieve much lower values.
Most wear environments have multiple points of contact and it is quite common that all mating surfaces are plated. The table below highlights the improved performance of electroless nickel when both mating surfaces are plated where the results are comparable to hard chrome.
is one of these. Electroless nickel is considered a very strong yet brittle material. The relatively low ductility is due to the various microcrystalline and amorphous structures that limit plastic
deformation, i.e the ability to “stretch” without fracture. It is not uncommon for heavy build deposits (in excess of 25 microns) to crack when exposed to severe loads. This is especially evident in lower phosphorus films or those plated from older baths (in excess of 150 g/l of orthophosphate) with high tensile stress.
Extrinsic stress is the least insidious and is brought on by differences in thermal coefficient of expansion between the deposit and the substrate. When the plated surfaces are first cooled there is an inherent shrinkage of the component. Electroless nickel typically shrinks roughly 0.1% on cooling from the operating bath temperature. When plated on substrates that have a higher COTE than EN (brass and aluminum for example) the EN deposit will develop tensile stress. On substrates that have a lower COTE than EN the deposit will be in a compressive state. This of course, assumes no loss of adhesion or serious cracking as the component seeks to adjust to the shrinkage.
Intrinsic stress is brought on by the plating process itself. Although the substrate also plays a small role, intrinsic stress is controlled by formation of the nickel layers due primarily to the EN formulation and bath operating conditions. A general remark to guide your thought process would be that higher phosphorus EN processes operated under good practice will produce deposits with neutral or compressive stress. In most applications, compressive stress is desired since it has a negligible effect on fatigue loss, improves adhesion and some have found improved corrosion protection. The graph below in Figure 12 reflects deposit stress vs phosphorus content. Note that lower phosphorus deposits (less than 4% b.w.) will also exhibit lower tensile stress. This can attributed to the presence of homogenous structural phases that Duncan reported years ago.
Assuming the proper EN formulation is selected to deliver the desired stress level, a reduction in compressive stress or even a shift to tensile is possible under certain conditions and must be avoided. Operating an EN bath outside of its recommend guidelines will impact stress. As a plater desires to push a process to plate faster, raising the pH is often the first button to push. When the pH is increased the deposit phosphorus drops, compressive stress is reduced and this can lead to negative results. Platers are commonly surprised to find a process that was plating with no stress cracks or adhesion failure the day before is now producing failures. A simple view of the record books or quick analysis reveals the culprit. An even more common contributor to an increase in an EN deposits tensile nature is bath age. It has been well documented that an EN process designed to produce a compressive deposit will stop doing so after a certain bath age (roughly 5 metal turnovers). It is at this point where the presence of reaction by products, sodium orthophosphate, begins to contribute to tensile stress. One can continue to plate in this bath but must be fully aware that stress will increase and cold lead to adhesion and/or cracking failures. This is most evident, again, with thicker films. Figure 13 represents this phenomenon. There are certain additives that, when used carefully, can reduce the tensile stress even as the EN bath ages.
The photos in figure 14 are of two identical parts, each plated in the same bath but at different bath ages. The part on the right was plated in a relatively new bath whereas the one on the left was plated in a bath nearly 10 metal turnovers old. You’ll note the clear evidence of flaking and separation due to the high tensile stress.
Armed with a better understanding of the properties of electroless nickel phosphorus deposits, both platers and engineers alike should be able to effectively select the proper EN process as well as necessary operating guides for each and every application they encounter. The following section provides an overview of these applications.
Differential pinion shafts and a variety of pins and washers are plated in large quantities due to the coatings lubricity, wear resistance and anti-galling properties (Figure 15). Brake pistons are plated in bright, medium phosphorus electroless nickel and heat treated to increase hardness and wear resistance. High deposit luster is desired and actually improves the surface finish of the piston resulting in reduced friction (Figure 16). Cast iron
slip yokes are plated with EN to eliminate noise associated with galling.
Future applications will most likely include the use of EN composites such as PTFE, boron nitride and silicon carbide. The use and growth of fuel cell technology in automobiles shows promise and electroless nickel could play a role. A challenge EN faced nearly a decade ago was the End-of Life Vehicle (ELV
) directive that bans the use of various toxic metals often found in EN deposits. Viewed as a barrier when it was first promulgated, many experts feel the ELV directive and other similar green initiatives have actually advanced EN technology.
substrates for magnetic data storage on computers. Uniformity, non-magnetic character and reliably smooth, defect free nature lend to its continued use. Over the past 20 years this application for EN has been threatened a number of times by emerging technology. In each case, EN has proven up to the challenge and maintained its position as the most reliable and cost effective technology. (Figure 17)
Heat sinks, semiconductor
packages, and battery components are examples of other high volume electroless nickel electronic applications (Figure 18). A variety of aluminium and zinc die cast connectors are plated with EN to enhance corrosion and wear resistance (Figure 19). Uniformity, electrical conductivity and solderability
are other important properties for this application.
An area of continued interest and growth is the plating of microwave components. With complex shapes and deep recessed areas electroless nickel is ideally suited to act as a corrosion resistant barrier film on top of aluminum and beneath the silver or tin electroplated topcoat.
Although certain technical barriers must be overcome, the use of medium phosphorus electroless nickel beneath immersion gold on circuit boards, shows promise. Commonly referred to as the ENIG (Electroless nickel immersion gold) process, it offers improved shelf life in humid conditions and maintains excellent solderability over periods of long storage.
Renewable energy also appears to offer promise for EN where its use for metalizing photovoltaic cells is developing quickly.
The following is a table that offers a general guide to selecting an electroless nickel for a specific application or requirement:
Phosphorus
Phosphorus is the chemical element that has the symbol P and atomic number 15. A multivalent nonmetal of the nitrogen group, phosphorus as a mineral is almost always present in its maximally oxidized state, as inorganic phosphate rocks...
deposits find the most use in the markets the plating
Plating
Plating is a surface covering in which a metal is deposited on a conductive surface. Plating has been done for hundreds of years, but it is also critical for modern technology...
industry serves.
Technology overview
Electroless nickel (EN) deposits are typically classified as functional coatings and historically have found use in applications that require protection from either corrosionCorrosion
Corrosion is the disintegration of an engineered material into its constituent atoms due to chemical reactions with its surroundings. In the most common use of the word, this means electrochemical oxidation of metals in reaction with an oxidant such as oxygen...
or wear and in some cases, both. Because of the unique deposit properties and uniformity of the resultant film, many other applications have emerged that continue to capitalize on the chameleon-like nature of electroless nickel. The majority of EN films used commercially are deposited from solutions formulated with sodium hypophosphite as the reducing agent. This results in nickel films that are alloyed with phosphorus in ranges between 1 and 12 weight percent.
The mechanical properties of NiP (ENP) deposits can be further enhanced not only by the co-deposition of inert particles such as teflon, silicon
Silicon
Silicon is a chemical element with the symbol Si and atomic number 14. A tetravalent metalloid, it is less reactive than its chemical analog carbon, the nonmetal directly above it in the periodic table, but more reactive than germanium, the metalloid directly below it in the table...
carbide or boron nitride
Boron nitride
Boron nitride is a chemical compound with chemical formula BN, consisting of equal numbers of boron and nitrogen atoms. BN is isoelectronic to a similarly structured carbon lattice and thus exists in various crystalline forms...
but also by alloying with a third element, forming a ternary alloy of NiPX, where X can be copper
Copper
Copper is a chemical element with the symbol Cu and atomic number 29. It is a ductile metal with very high thermal and electrical conductivity. Pure copper is soft and malleable; an exposed surface has a reddish-orange tarnish...
, tungsten
Tungsten
Tungsten , also known as wolfram , is a chemical element with the chemical symbol W and atomic number 74.A hard, rare metal under standard conditions when uncombined, tungsten is found naturally on Earth only in chemical compounds. It was identified as a new element in 1781, and first isolated as...
, molybdenum
Molybdenum
Molybdenum , is a Group 6 chemical element with the symbol Mo and atomic number 42. The name is from Neo-Latin Molybdaenum, from Ancient Greek , meaning lead, itself proposed as a loanword from Anatolian Luvian and Lydian languages, since its ores were confused with lead ores...
or tin depending on the particular formulation.
Electroless nickel boron (NiB) alloys are also well cited in the literature, although they are less commercially viable than the NiP alloys. The films are generated using either sodium borohydride or dimethylaminoborane as the reducing agent and can range in boron content from 1–5 weight percent. NiB films are typically used in the electronics industry where low-resistivity coatings are required and also find use in industrial applications when extreme wear and increased coating hardness is specified.
Electroless nickel is no different than its electroplated counterparts in that it is only as strong as the weakest link in the process cycle. More clearly stated, successful electroless nickel plating
Electroless nickel plating
Electroless nickel plating is an auto-catalytic chemical technique used to deposit a layer of nickel-phosphorus or nickel-boron alloy on a solid workpiece, such as metal or plastic. The process relies on the presence of a reducing agent, for example hydrated sodium hypophosphite which reacts with...
requires both strict adherence to bath operating guidelines and optimal surface preparation. A well formulated electroless nickel process will not overcome improper surface preparation. Conversely, applying the wrong electroless nickel will short-circuit success even when the substrate is wonderfully prepared. The following links are critical:
- Proper surface preparation
- Choice of electroless nickel
- Conformance to operating guidelines.
An inability to recognize the interconnectivity of these three basic items will ultimately lead to failure somewhere along the supply line, hopefully, before the component heads to the field.
Microstructure and composition of ENP Films
One of the distinct advantages of the electroless nickel deposition process is the ability to produce an alloy of nickel and phosphorus in varying composition. Depending on the formulation and the operation of the chemistry the film compositions can vary from 2 to 13 weight percent phosphorus. This variation in alloy content has a significant affect on the deposit microstructure and performance characteristics and offers flexibility to well informed platers and engineers that can take full advantage of these differences.Electrodeposited nickel has a purity of greater than 99% and is highly crystalline
Crystallinity
Crystallinity refers to the degree of structural order in a solid. In a crystal, the atoms or molecules are arranged in a regular, periodic manner. The degree of crystallinity has a big influence on hardness, density, transparency and diffusion. In a gas, the relative positions of the atoms or...
. On the other hand, electroless nickel deposits that contain more than 10.5% phosphorus appear to be amorphous
Amorphous solid
In condensed matter physics, an amorphous or non-crystalline solid is a solid that lacks the long-range order characteristic of a crystal....
, i.e., lacking crystal structure. EN deposits with less than 7 weight percent phosphorus have a clear microcrystalline structure (2-6 nm grain size) and film properties are distinctly different. Some studies have found that higher phosphorus deposits (above 10.5 weight percent phosphorus) may not be truly amorphous but rather a mixture of microcrystalline and amorphous phases.
Putting aside some of the well known facts about deposit phosphorus vs various properties, i.e hardness or corrosion resistance that we will cover a bit later, deposits with a high degree of amorphous composition are free of grain boundaries, which typically act as sites for intergranular corrosion commonly encountered in crystalline deposits. There are also applications that benefit from crystalline structure as well. Duncan pointed out that in the as deposited condition an electroless nickel coating can contain both crystalline and amorphous phases or, if dictated by formula, may only contain one. Each phase offers distinct benefits and understanding what exists in the deposit you plan to apply is relevant.
Deposit uniformity
A significant advantage of the electroless nickel process is the ability to produce deposits with uniform thickness on parts with complex geometries and shapes. Since this is a chemical reaction, any catalytic surface exposed to the plating solution will plate uniformly, provided it meets the criteria established a few paragraphs earlier. The current density affects typically associated with electroplatingElectroplating
Electroplating is a plating process in which metal ions in a solution are moved by an electric field to coat an electrode. The process uses electrical current to reduce cations of a desired material from a solution and coat a conductive object with a thin layer of the material, such as a metal...
are not a factor, therefore sharp edges, deep recesses and blind holes are readily plated to uniform thickness with electroless nickel chemistry. Many applications for electroless nickel exist today because it is often the only way to plate certain components. The difference in deposit uniformity is illustrated in figure 1. Figure 2 is a photo of EN plated bumps on a flip chip, something impossible to accomplish with an electroplated deposit.
The degree of uniformity can vary on edges, threads, small holes or deep recesses where exchange of fresh solution may be difficult. This can also occur under conditions with excessive bath agitation, especially in the presence of heavy metals. This thickness variation may be controlled by optimizing solution dynamics and/or by controlling the concentration of certain additives formulated into the EN plating bath.
Melting point
Unlike electroplated nickel, electroless nickel deposits do not have a precise melting point but rather have a melting range. Pure nickel has a melting point of 1455°C (2650°F), however EN is an alloy and as the phosphorus content is increased within the film, the deposit begins to soften at lower temperatures and continues to soften until it eventually melts. The melting range decreases linearly as the phosphorus levels increase. The eutectic or lowest melting point for NiP alloys is 880°C (1620°F) and occurs at a deposit phosphorus content of 11% b.w. The highest melting point is for low Phosphorus deposits (less than 3%) that claim to have melting points at 1,200°C (2,200°F). EN for some high temperature applications have failed since it was not fully recognized that even with that high of a range the deposit still will have liquid phase at much lower temperatures. Figure 3 illustrates what happens when you subject EN to temperatures above its melting point.Electrical resistivity
The electrical resistivity of electroless nickel alloys is higher than that of pure nickel. High purity nickel has a specific resistivity of 7.8 x 10-6 ohm-cm. Increasing the phosphorus content increases the electrical resistivity of the film. (Figure 4.) Values range from 30-100 x 10-6 ohm-cm. Heat treatment of the ENP film can affect resistivity. At temperatures as low as 150°C resistivity will decrease due to release of physically absorbed hydrogen. At temperatures greater than 250°C a similar marked decrease will occur as a result of phosphorus migration and the structural transformation to Nickel Phosphide.Magnetic properties
The single largest application for electroless nickel is as a sub layer for computer memory discs. To meet the requirements for this application the coating must remain non-magnetic even after one-hour bake cycles of 250-300°C. In recent years, the data storage industry has pushed the high side of this temperature range even higher. This can be achieved only with high phosphorus alloys (> 10.5% P) and/or with specific additives. The non-magnetic property of these high phosphorus films is one of the most important physical characteristics.Figure 5 depicts the effect phosphorus content has on ferromagnetic properties.
Not all high phosphorus alloys will maintain the same level of thermomagnetic stability. The deposit performance is also dependent on the chemical formulation and the solution dynamics during the plating process. Reducing the onset and the rate of crystallization, minimizing the volume fraction of microcrystallinity and maintaining a homogeneous grain structure at higher bake temperatures are key requirements for optimization of the films thermomagnetic performance.
Corrosion resistance
The most widespread application of electroless nickel technology is to provide superior corrosion protection in a multitude of corrosive environments. Market data clearly indicates that the majority of applications for EN are on steel and aluminium substrates that are anodic or less noble than EN. EN acts as a barrier coating that protects by providing a pore free barrier coating against the corrosive environment. This is in direct contrast to sacrificial films such as zincZinc
Zinc , or spelter , is a metallic chemical element; it has the symbol Zn and atomic number 30. It is the first element in group 12 of the periodic table. Zinc is, in some respects, chemically similar to magnesium, because its ion is of similar size and its only common oxidation state is +2...
or zinc alloy
Zinc alloy
Electroplated Zinc alloys have been developed for meeting the most challenging specifications in terms of corrosion protection, temperature and wear resistance.-History:...
that protect the base material by sacrificing itself.
The nature of the corrosive environment and the deposits resistance to chemical attack are also important criteria when selecting a particular EN coating for optimum performance. High phosphorus coatings (10-12%) are more readily attacked in strongly alkaline media than low phosphorus deposits (1-3%), however they perform significantly better in acidic media. This is illustrated in the following graphs.
Optimizing the performance of EN coatings for maximum corrosion protection requires that the coating be continuous and free of any microporosity, roughness, nodules and irregularities within the microstructure. Since the microstructure of high phosphorus EN films are amorphous they are essentially free of the grain boundaries that could potentially serve as sites for corrosion. The absence of such phase boundaries and the ability of the high phosphorus EN film to form a passive film on its surface, make it an excellent choice for protection on aluminum and steel substrates in highly corrosive, acidic environments.
The phosphorus content alone however is not enough to maximize the coatings corrosion resistance in a given environment. Factors that will affect the corrosion performance of EN films are:
- 1.Proper selection of the EN process and deposit thickness
- 2.Operation and maintenance of the process, minimizing co-deposited impurities
- 3.Minimizing substrate defects through improved OEM training on substrate selection (cast vs extruded) and various pre-plate mechanical operations (forming, stamping, grinding, etc)
- 4.Selecting the optimum pretreatment cycle
- 5.Post plate operations (both good and bad). Bad- High temperature post treatment that can produce micro-cracked deposits-Bad. Good-Post dips, low temperature bakes, etc
Surface condition plays an important role in determining the required deposit thickness and good field performance. For example, machined substrates alloyed with sulfur and/or lead are particularly vulnerable to a high degree of porosity, since these alloying constituents are catalytic poisons to the deposition process. Substrates with high roughness values require thicker deposits to provide adequate corrosion protection. This is primarily due to the formation of nodules on the roughened during the initial stages of deposition, resulting in microporosity of the ENP film.
Increasing the deposit thickness can minimize this affect. Smooth substrates require a thickness of 25 microns to obtain a film with minimal porosity whereas roughened surfaces can require up to three times the thickness to provide similar corrosion protection.
The use of neutral salt spray, in accordance with the ASTM B 117 specification, for the measurement of corrosion performance is well documented in the literature and is still the most widely accepted method for coating evaluation. The results of neutral salt spray exposure, phosphorus content and deposit thickness are summarized in the table below:
Coating Thickness | 4-5% ENP | 7-8% ENP | 6.5-8% ENP | 10.5-12.0% ENP |
---|---|---|---|---|
12 microns (0.4 mils) | 24 | 24 | 24 | 250 |
22 microns (0.8 mils) | 96 | 96 | 96 | 1,000 |
38 microns (1.4 mils) | 96 | 96 | 96 | 1,000 |
50 microns (2.0 mils) | 96 | 96 | 96 | 1,000 |
Deposit hardness
Deposit hardness is one of the key tribological properties of ENP coating technology. Factors that affect hardness are the film composition (%P), the heat treatment temperature and the heat treatment time. Typical microhardness values for the as plated ENP deposits range from 500 to 720 VHN (Figure 8.) This is in contrast to electrolytically deposited nickel, which have typical values of 150-400VHN.Heat treatment of ENP deposit will significantly increase the microhardness. Figure 9 illustrates the effective temperature range and time required to attain specific values.
In cases where the substrate cannot withstand the temperature requirements to achieve maximum hardness, low phosphorus EN is often recommended. The increase in microhardness for ENP films is attributed to the phase transformations that take place during the heat cycle to form nickel metal and nickel phosphide. Some volume shrinkage and cracking of the film will occur and must be taken into account if the application requires corrosion protection.
Wear resistance
Electroless Nickel Phosphorus coatings are specified for a wide variety of engineered applications related to wear resistance. This is mainly attributed to the fact that the coatings not only have high hardness and intrinsic lubricity but also afford excellent corrosion resistance and deposit uniformity. The wear properties of ENP films can also be enhanced through the codeposition of inert particles such as PTFE, Silicon Carbide or Boron Nitride forming a composite coating.The abrasive wear of ENP films are typically measured by applying a mechanical action of an abrasive, rotating wheel on the plated surface and measuring the weight loss (in milligrams) of the coating at intervals of 1000 cycles. This test is referred to as the Taber Wear Index (TWI) and perhaps is the most frequently used method to evaluate the wear characteristics of ENP films. Typical weight loss results for various deposits are illustrated in Figure 10.
The “as plated” low phosphorus deposits tend to withstand abrasive wear better than the high phosphorus coatings, largely attributed to the higher hardness values. However after heat treatment the trends remain the same even though the deposit hardness is similar suggesting that other factors, such as deposit composition, play a role in abrasive wear resistance.
Adhesive wear is defined as the removal of material between mating surfaces and measures the films ability to resist galling, welding or seizing. If the mating surfaces are both electroless nickel, optimum performance is achieved if the surfaces in contact have dissimilar properties, such as phosphorus content or hardness. The adhesive wear resistance of ENP films improves with increasing phosphorus content.
It is important to consider as many variables as possible when evaluating an ENP coating for tribological applications. Factors such as hardness, phosphorus content, the presence of corrosive liqiuids or gases, the temperature, the nature of the mating surface, the degree of lubrication and the characteristics of the substrate are critical to optimum performance.
Lubricity
As pointed out above, lubricity plays an important role in optimizing wear resistance. It does so by inhibiting contact between mating surfaces, reducing friction and associated heat. Hard chromeChromium
Chromium is a chemical element which has the symbol Cr and atomic number 24. It is the first element in Group 6. It is a steely-gray, lustrous, hard metal that takes a high polish and has a high melting point. It is also odorless, tasteless, and malleable...
is well known as possessing a self healing lubricating film. Whereas EN does not exhibit this feature it still offers excellent lubricity
Lubricity
Lubricity is the measure of the reduction in friction of a lubricant. The study of lubrication and mechanism wear is called tribology.-Measurement of lubricity:...
and finds many uses that can capitalize on this property. Figure 11 graphically displays the coefficient of friction vs. non lubricated steel
Steel
Steel is an alloy that consists mostly of iron and has a carbon content between 0.2% and 2.1% by weight, depending on the grade. Carbon is the most common alloying material for iron, but various other alloying elements are used, such as manganese, chromium, vanadium, and tungsten...
. Hard chrome does perform better than standard high phosphorus EN (HP EN) but with occlusion of various soft particles you can achieve much lower values.
Most wear environments have multiple points of contact and it is quite common that all mating surfaces are plated. The table below highlights the improved performance of electroless nickel when both mating surfaces are plated where the results are comparable to hard chrome.
Mating Surface | Coefficient of Friction | |
Unlubricated | Lubricated | |
Electroless nickel vs Steel | 0.38 | 0.20 |
Chrome vs Steel | 0.21 | 0.13 |
Steel vs Steel | galling | 0.2 |
Chrome vs Chrome | 0.43 | 0.26 |
EN vs EN | 0.45 | 0.20 |
Elecroless nickel vs Chrome | 0.43 | 0.30 |
EN PTFE vs steel | 0.12 | |
EN Boron nitride vs steel | 0.07 |
Ductility
Although electroless nickel is widely considered an engineering coating it does not compare well to other coatings in terms of certain mechanical properties. DuctilityDuctility
In materials science, ductility is a solid material's ability to deform under tensile stress; this is often characterized by the material's ability to be stretched into a wire. Malleability, a similar property, is a material's ability to deform under compressive stress; this is often characterized...
is one of these. Electroless nickel is considered a very strong yet brittle material. The relatively low ductility is due to the various microcrystalline and amorphous structures that limit plastic
Plastic
A plastic material is any of a wide range of synthetic or semi-synthetic organic solids used in the manufacture of industrial products. Plastics are typically polymers of high molecular mass, and may contain other substances to improve performance and/or reduce production costs...
deformation, i.e the ability to “stretch” without fracture. It is not uncommon for heavy build deposits (in excess of 25 microns) to crack when exposed to severe loads. This is especially evident in lower phosphorus films or those plated from older baths (in excess of 150 g/l of orthophosphate) with high tensile stress.
Internal stress
Internal stress is arguably one of the most important mechanical properties of electroless nickel and often the most ignored. Internal stress plays a role in adhesion, wear and corrosion resistance and therefore must be regulated or at a minimum, taken notice of. Internal stress can be developed both extrinsically and intrinsically.Extrinsic stress is the least insidious and is brought on by differences in thermal coefficient of expansion between the deposit and the substrate. When the plated surfaces are first cooled there is an inherent shrinkage of the component. Electroless nickel typically shrinks roughly 0.1% on cooling from the operating bath temperature. When plated on substrates that have a higher COTE than EN (brass and aluminum for example) the EN deposit will develop tensile stress. On substrates that have a lower COTE than EN the deposit will be in a compressive state. This of course, assumes no loss of adhesion or serious cracking as the component seeks to adjust to the shrinkage.
Intrinsic stress is brought on by the plating process itself. Although the substrate also plays a small role, intrinsic stress is controlled by formation of the nickel layers due primarily to the EN formulation and bath operating conditions. A general remark to guide your thought process would be that higher phosphorus EN processes operated under good practice will produce deposits with neutral or compressive stress. In most applications, compressive stress is desired since it has a negligible effect on fatigue loss, improves adhesion and some have found improved corrosion protection. The graph below in Figure 12 reflects deposit stress vs phosphorus content. Note that lower phosphorus deposits (less than 4% b.w.) will also exhibit lower tensile stress. This can attributed to the presence of homogenous structural phases that Duncan reported years ago.
Assuming the proper EN formulation is selected to deliver the desired stress level, a reduction in compressive stress or even a shift to tensile is possible under certain conditions and must be avoided. Operating an EN bath outside of its recommend guidelines will impact stress. As a plater desires to push a process to plate faster, raising the pH is often the first button to push. When the pH is increased the deposit phosphorus drops, compressive stress is reduced and this can lead to negative results. Platers are commonly surprised to find a process that was plating with no stress cracks or adhesion failure the day before is now producing failures. A simple view of the record books or quick analysis reveals the culprit. An even more common contributor to an increase in an EN deposits tensile nature is bath age. It has been well documented that an EN process designed to produce a compressive deposit will stop doing so after a certain bath age (roughly 5 metal turnovers). It is at this point where the presence of reaction by products, sodium orthophosphate, begins to contribute to tensile stress. One can continue to plate in this bath but must be fully aware that stress will increase and cold lead to adhesion and/or cracking failures. This is most evident, again, with thicker films. Figure 13 represents this phenomenon. There are certain additives that, when used carefully, can reduce the tensile stress even as the EN bath ages.
The photos in figure 14 are of two identical parts, each plated in the same bath but at different bath ages. The part on the right was plated in a relatively new bath whereas the one on the left was plated in a bath nearly 10 metal turnovers old. You’ll note the clear evidence of flaking and separation due to the high tensile stress.
Armed with a better understanding of the properties of electroless nickel phosphorus deposits, both platers and engineers alike should be able to effectively select the proper EN process as well as necessary operating guides for each and every application they encounter. The following section provides an overview of these applications.
Applications
Electroless nickel gained market acceptance over the past 50 years through a combination of trial and errors, excellent marketing initiatives and commitment from many plating shop pioneers willing to take on a fledgling technology. Today, electroless nickel offers the engineering community a technology that is as reliable as it is diverse in meeting various application challenges. An approximate breakdown of worldwide applications for electroless nickel is detailed below:Automotive
Applications for use in this industry take full advantage of the many benefits electroless nickel has to offer. Deposit properties such as uniformity, corrosion resistance, lubricity and wear resistance are all reasons why the use of EN for automotive applications continues to grow. Historically, a cost sensitive market segment, auto makers have embraced the use low cost materials plated with a thin film of electroless nickel to meet strict requirements in an economical fashion. Fuel injection systems, aluminum fuel filters, brake pins and bleed valves are just a few applications that take full advantage of the deposit’s corrosion resistance.Differential pinion shafts and a variety of pins and washers are plated in large quantities due to the coatings lubricity, wear resistance and anti-galling properties (Figure 15). Brake pistons are plated in bright, medium phosphorus electroless nickel and heat treated to increase hardness and wear resistance. High deposit luster is desired and actually improves the surface finish of the piston resulting in reduced friction (Figure 16). Cast iron
Iron
Iron is a chemical element with the symbol Fe and atomic number 26. It is a metal in the first transition series. It is the most common element forming the planet Earth as a whole, forming much of Earth's outer and inner core. It is the fourth most common element in the Earth's crust...
slip yokes are plated with EN to eliminate noise associated with galling.
Future applications will most likely include the use of EN composites such as PTFE, boron nitride and silicon carbide. The use and growth of fuel cell technology in automobiles shows promise and electroless nickel could play a role. A challenge EN faced nearly a decade ago was the End-of Life Vehicle (ELV
End of Life Vehicles Directive
The End of Life Vehicles Directive is a Directive of the European Union addressing the end of life for automotive products. Every year, motor vehicles which have reached the end of their useful lives create between 8 and 9 million tonnes of waste in the European Union...
) directive that bans the use of various toxic metals often found in EN deposits. Viewed as a barrier when it was first promulgated, many experts feel the ELV directive and other similar green initiatives have actually advanced EN technology.
Aerospace
Design engineers have found the combination of electroless nickel’s functional properties to be very appealing for aerospace use. Due to the obvious reliability issues associated with this market segment, a long term, thorough evaluation of electroless nickel has been on going and had made for slow progress. Successful testing and application for a number of years resulted in a better understanding of when and how to specify electroless nickel. It’s use on engine assemblies, servo valves, landing gear, turbine blades and the like have found widespread use. Unlike hard chrome, compressive stressed high phosphorus deposits do not significantly reduce the fatigue strength of these critical components. For this reason and reasons stated above, its continued application appears secure.Electronics
Use of electroless nickel for electronic applications continues to grow and is clearly the most diverse market segment. Magnetic properties, corrosion resistance and solderability are properties that have contributed the most to its success. A large application involves the use of high phosphorus deposits on polished aluminiumAluminium
Aluminium or aluminum is a silvery white member of the boron group of chemical elements. It has the symbol Al, and its atomic number is 13. It is not soluble in water under normal circumstances....
substrates for magnetic data storage on computers. Uniformity, non-magnetic character and reliably smooth, defect free nature lend to its continued use. Over the past 20 years this application for EN has been threatened a number of times by emerging technology. In each case, EN has proven up to the challenge and maintained its position as the most reliable and cost effective technology. (Figure 17)
Heat sinks, semiconductor
Semiconductor
A semiconductor is a material with electrical conductivity due to electron flow intermediate in magnitude between that of a conductor and an insulator. This means a conductivity roughly in the range of 103 to 10−8 siemens per centimeter...
packages, and battery components are examples of other high volume electroless nickel electronic applications (Figure 18). A variety of aluminium and zinc die cast connectors are plated with EN to enhance corrosion and wear resistance (Figure 19). Uniformity, electrical conductivity and solderability
Solderability
The solderability of a substrate is a measure of the ease with which a soldered joint can be made to that material. Good solderability requires wetting of the substrate by the solder....
are other important properties for this application.
An area of continued interest and growth is the plating of microwave components. With complex shapes and deep recessed areas electroless nickel is ideally suited to act as a corrosion resistant barrier film on top of aluminum and beneath the silver or tin electroplated topcoat.
Although certain technical barriers must be overcome, the use of medium phosphorus electroless nickel beneath immersion gold on circuit boards, shows promise. Commonly referred to as the ENIG (Electroless nickel immersion gold) process, it offers improved shelf life in humid conditions and maintains excellent solderability over periods of long storage.
Renewable energy also appears to offer promise for EN where its use for metalizing photovoltaic cells is developing quickly.
Chemical processing
Requirements for this application often include the need to maintain product purity in addition to the typical needs for uniformity and corrosion resistance. Selecting the proper electroless nickel is often critical to successful application. Recent studies have found phosphorus content plays an important role in the overall performance of the EN deposit in a specific environment. Pumps, valves and flanges are typically plated with 50-100 microns of electroless nickel for very severe applications.Oil and gas industry
A proving ground for electroless nickel over the past 25 years, the success of electroless nickel for these applications is well documented. Ball valves, heat exchangers, pumps, etc fabricated from less expensive materials and plated with high phosphorus EN has greatly contributed to the success. Corrosion resistance in harsh environments and resistance to erosive wear as well as its uniformity will allow EN to maintain its dominant position in this market segment.Other applications
Food handling, mold protection, foundry tooling, plating on non-conductors and the printing industry are a few other applications where electroless nickel finds significant use. Textile applications (Figure 20) utilize excellent wear resistance and lubricity characteristics. Composite coatings such as EN/boron nitride have found increased use in applications that require extending service life (Figure 21).The following is a table that offers a general guide to selecting an electroless nickel for a specific application or requirement:
Application or desired deposit characteristic |
Low P (1-3% b.w.) |
Low-mid P (4-6% b.w.) |
Medium P (7-9% b.w.) |
High P (10.5-12% b.w.) |
EN-PTFE EN/Boron nitride |
EN-SiCarbide EN-Diamond |
---|---|---|---|---|---|---|
Wear resistance-moderate | ▲ | ▲ | ▲ | ▲ | ▲ | |
Wear resistance-severe | ▲ | |||||
Corrosion resistance per ASTM B117 |
▲ | |||||
Solderability* | ▲ | ▲ | ||||
High temperature exposure | ▲ | |||||
Release/low friction | ▲ | |||||
Chemical resistance (acid) | ▲ | |||||
Chemical resistance (alkaline) | ▲ | ▲ | ||||
Brightness | ▲ | ▲ | ||||
Heavy build/diamond turning | ▲ | |||||
Non-Magnetic property | ▲ |