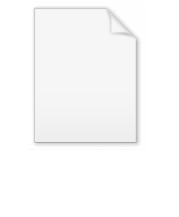
Desulfonylation reactions
Encyclopedia
Desulfonylation reactions are chemical reactions leading to the removal of a sulfonyl group from organic compounds. As the sulfonyl functional group is electron-withdrawing, methods for cleaving the sulfur-carbon bonds of sulfones are typically reductive in nature. Olefination or replacement with hydrogen may be accomplished using reductive desulfonylation methods.
functional group (RS(O)2R') has become an important electron-withdrawing group for modern organic chemistry. α-Sulfonyl carbanions may be used as nucleophiles in alkylation reactions, Michael-type additions, and other processes. After having served their synthetic purpose, sulfonyl groups are often removed. In the presence of certain reducing agents, one of the sulfur-carbon bonds of the sulfonyl group is cleaved, leading to sulfur-free organic products. Depending on the nature of the substrate and reaction conditions, alkyl sulfones afford either the corresponding alkanes or olefins (the Julia olefination
). Reductive desulfonylation is typically accomplished with active metals or salts (sodium amalgam
, aluminium amalgam, magnesium
, samarium(II) iodide
), tin hydrides (tributyltin hydride
), or transition metal complexes with reducing agents or nucleophiles (PdCl2(dppp)/LiHBEt3, Pd(PPh3)4
/LiHBEt3, Pd(PPh3)4
/NaHC(CO2Et)2). Alkyl, alkenyl, and allylic sulfones may be reduced using one or more of these methods.
(1)
(2)
Samarium(II) iodide may be used to reductively cleave α-keto sulfones; in the presence of hexamethylphosphoramide (HMPA), SmI2 is able to effect reductive elimination of α-functionalized sulfones (see equation (11) below).
(3)
Tin hydrides reduce α-keto and allylic sulfones. The mechanisms of these processes involve the addition of a tin-centered radical to the substrate followed by elimination of a sulfinyl radical, which abstracts a hydrogen from a molecule of tin hydride to propagate the radical chain. Protonation of the organotin intermediates thus formed (by sulfinic acid generated in situ) leads to reduced products. Addition of a stoichiometric amount of proton source allows the use of tin hydride in catalytic amounts. Although desulfonylations of allylic sulfones are site selective (providing only products of allylic transposition), they are not stereoselective and afford mixtures of double bond isomers. The mechanism of desulfonylation of α-keto sulfones is similar.
(4)
Transition-metal-mediated reductive desulfonylations rely on the generation of an intermediate π-allyl complex, which undergoes nucleophilic attack by hydride or another nucleophile to afford reduced products. Nucleophilic attack generally occurs at the less substituted position position of the π-allyl moiety, although site selectivity depends strongly on the substrate and reaction conditions. Palladium(0) complexes are the most commonly used precatalysts.
(5)
, which yields alkenes via addition of an α-sulfonyl carbanion to an aldehyde followed by reductive elimination. Sodium amalgam or samarium(II) iodide/HMPA may be used to convert β-sulfonyloxy or β-acyloxy sulfones to the corresponding alkenes. The key mechanistic step of this process is elimination of an anionic or organometallic intermediate to generate the alkene.
(6)
The use of sodium amalgam, which promotes the formation of essentially free alkyl anions, leads to (E) alkenes with extremely high selectivity. Samarium(II) iodide also produces the (E) alkene predominantly, but with lower selectivity.
Alkyl sulfones may be reduced with sodium or lithium in liquid ammonia; however, the strongly basic conditions of these dissolving metal reductions represent a significant disadvantage. In alcoholic solvents, magnesium metal and a catalytic amount of mercury(II) chloride may be used. A wide variety of functional groups are unaffected by these conditions, including many that are transformed by dissolving metal reductions. Reductive desulfonylation with these reagents does not occur in reactions of β-hydroxy sulfones, due to the poor leaving group ability of the hydroxyl group.
(7)
A significant issue associated with the reduction of allylic sulfones is transposition of the allylic double bond, which occurs in varying amounts during reductions by metal amalgams. and tin hydrides Palladium-catalyzed reductive desulfonylations of allylic sulfones do not have this issue, and afford allylic sulfones with high site and stereoselectivity.
(8)
Aluminum amalgam (Al/Hg) may be used for the chemoselective reduction of α-sulfonylated carbonyl groups. Carboxylic acid derivatives, acetals, thioacetals, amines, alcohols, and isolated double bonds are all inert to Al/Hg. Selective desulfonylation may be carried out on β-hydroxy sulfones without reductive elimination.
(9)
Transition metal catalysis is also useful for the stereospecific reduction of alkenyl sulfones. In the presence of an excess of a Grignard reagent, a palladium(II) or nickel(II) catalyst, and a phosphorus or nitrogen ligand, alkenyl sulfones are converted to the corresponding alkenes stereospecifically in good yield. On the other hand, dissolving metal and metal amalgam reductions are not stereoselective in general. Palladium catalysis is generally superior to nickel catalysis, giving higher yields and stereoselectivities.
(10)
Alkyl and alkenyl sulfones with good leaving groups in the β position undergo elimination under reductive conditions to afford alkenes or alkynes. The Julia olefination
exploits this process for the synthesis of alkenes from alkyl sulfones and carbonyl compounds. Addition of an α-sulfonyl anion to a carbonyl compound, followed by quenching with an acyl or sulfonyl chloride, leads to a β-acyloxy or -sulfonyloxy sulfone, which undergoes elimination under reductive conditions. Sodium amalgam may be used to accomplish the elimination step; however, the combination of samarium(II) iodide and HMPA is milder than strongly basic sodium amalgam and leads to higher yields in reductive elimination processes.
(11)
(12)
Reductive desulfonylation is employed when the establishment of a carbon-carbon single bond is the goal. In a synthesis of (+)-chatancin, alkylation of an α-sulfonyl carbanion followed by desulfonylation established a key allylic carbon-carbon bond.
(13)
(14)
A wide variety of carbonyl olefination methods that are direct alternative to the Julia olefination are known: the Wittig reaction
, the Horner-Wadsworth-Emmons reaction
, Peterson olefination
, and others. The primary advantage of Julia olefination is that the sulfone precursors are sometimes more readily available and easier to purify than the corresponding phosphorus- or silicon-containing compounds. Additionally, a variety of methods to synthesize sulfones exist. Nonetheless, the sometimes limited stereoselectivity (and in particular, the difficulty of accessing (Z)-alkenes) of the Julia reaction can be problematic. Many alternative methods for olefination, including the Peterson reaction, do not have this issue.
(15)
Reductions employing an excess of alkali metals in ammonia or amines are extremely rapid and should be quenched after a minute or so of reaction time—longer reaction times promote side reactions. When lithium or sodium in ammonia is used, the persistance of a deep blue color in the solution (due to solvated electrons) is a sign that the reaction is complete. Reductions employing alkali metal arene radical anion complexes similarly require a large excess of the reducing agent, very low temperatures and short reaction times. Reductive desulfonylations and reductive eliminations employing alkali or alkaline earth metals in ammonia present a high fire hazard and should be conducted in a properly functioning chemical fume hood away from flammable solvents. Ammonia is a corrosive gas with a pungent odor. Alkali metals react violently with water or even moist air to generate hydrogen, which can then be ignited by the heat of the reaction.
In pure alcoholic solvents, magnesium metal is the most commonly employed reducing agent. The required reaction temperature varies substantially across different substrates, although room temperature is usually preferred.
Sodium amalgam containing 2–6% sodium (prepared by the treatment of ribbons of sodium metal with mercury(0)) is the most commonly employed reagent for the reductive desulfonylation and reductive elimination processes. Disodium hydrogen phosphate is used to buffer the solution at a pH of 7–8, meaning that base-labile functionality is stable under the reduction conditions. Reductions employing aluminum amalgam (prepared by immersion of aluminum foil into a 2% aqueous solution of HgCl2) often require higher temperatures than the corresponding sodium amalgam reactions. Mercury and mercury compounds are poisonous and teratogenic, and should be used only in a properly functioning chemical fume hood. Mercury cannot be destroyed but it can be removed from aqueous solutions by using ion-exchange resins or by amalgamation with iron.
Samarium(II) iodide may be easily prepared by the reduction of samarium metal with organic dihalides in THF. It is most often used directly as a solution in THF. Hexamethylphosphoramide increases the reducing power of SmI2 substantially.
Tin halide reductions are almost always accomplished with tributyltin hydride and azobis(isobutyronitrile) (AIBN) as a radical initiator. Tin hydrides are toxic irritants and should be handled with care in a fume hood.
Among transition-metal-mediated reactions, the use of [PdCl2(dppp)] and superhydride (LiHBEt3) at low temperatures is preferred for reductions of allylic sulfones. Lithium triethylborohydride is supplied as a 1 M solution in tetrahydrofuran
, is corrosive and flammable, and should be stored under an inert atmosphere in a cool, dry place. Vinylic sulfones may be reduced with palladium(II) catalysts such as [Pd(acac)2] and Grignard reagents in tetrahydrofuran at room temperature. Tetrahydrofuran solutions of n-BuMgCl are highly flammable, sensitive to moisture, and cause burns. Homogeneous organonickel complexes, used most often in excess in THF at room temperature, are used to reduce alkyl sulfones.
Argon was bubbled into a solution of (S, E)-methyl 2-benzamido-4-fluoro-2-methyl-4-(phenylsulfonyl)but-3-enoate (41 mg, 0.10 mmol) in benzene (1 mL) for 2 minutes. Tributyltin hydride (64 mg, 0.20 mmol) and AIBN (2.0 mg, 0.01 mmol) were then added under an argon atmosphere. The reaction mixture was heated under reflux for 24 hours, concentrated, and the residue was chromatographed (hexane to 90:10 hexane/EtOAc) to give the tri-n-butylstannyl vinylalaninate derivative (45 mg, 80%): IR (film) 1741, 1525 cm–1; 1H NMR (500 MHz, CDCl3) δ 0.85 (m, 9H), 0.99 (m, 6H), 1.30 (m, 6H), 1.50 (m, 6H), 1.81 (s, 3H), 3.78 (s, 3H), 5.38 (d, J = 56.0 Hz, 1H), 7.40 (m, 2H), 7.46 (d, J = 7.0 Hz, 1H), 7.49 (bs, 1H), 7.77 (m, 2H); 13C NMR 125 MHz, (CDCl3) δ 8.7, 10.1, 13.5, 13.6, 24.3, 26.8, 27.0, 27.2, 28.6, 28.7, 28.8, 52.9, 57.9, 58.0, 124.2, 127.0, 127.1, 128.4, 131.4, 134.6, 165.9, 172.2, 173.9, 174.7; 19F NMR (470 MHz, CDCl3) δ –94.99 (d, J = 55.0 Hz). Anal. Calcd for C25H40NO3FSn: C, 55.58; H, 7.46; N, 2.59. Found: C, 54.85; H, 7.34; N, 2.54.
A suspension of the tri-n-butylstannyl vinylalaninate (46.4 mg, 0.1 mmol) in 6 N HCl (2 mL) was refluxed for 17 hours. Following sequential extraction with CH2Cl2 and EtOAc, the aqueous layer was evaporated under vacuum and mild heating (40°) to give the title product salt (13.4 mg, 89%): 1H NMR (500 MHz, D2O) δ 1.73 (s, 3H), 5.21 (dd, J = 44.0, 5.0 Hz, 1H), 6.77 (dd, J = 82.0, 5.0 Hz, 1H); 19F NMR (470 MHz, CDCl3) δ –117.85 (dd, J = 82.0, 43.0 Hz); HRMS–FAB (m/z): [M + H]+ calcd for C5H9FNO2,134.0617; found, 134.0616.
Introduction
The sulfonylSulfonyl
A sulfonyl group can refer either to a functional group found primarily in sulfones or to a substituent obtained from a sulfonic acid by the removal of the hydroxyl group similarly to acyl groups...
functional group (RS(O)2R') has become an important electron-withdrawing group for modern organic chemistry. α-Sulfonyl carbanions may be used as nucleophiles in alkylation reactions, Michael-type additions, and other processes. After having served their synthetic purpose, sulfonyl groups are often removed. In the presence of certain reducing agents, one of the sulfur-carbon bonds of the sulfonyl group is cleaved, leading to sulfur-free organic products. Depending on the nature of the substrate and reaction conditions, alkyl sulfones afford either the corresponding alkanes or olefins (the Julia olefination
Julia olefination
The Julia olefination is the chemical reaction of phenyl sulfones with aldehydes to give alkenes after alcohol functionalization and reductive elimination using sodium amalgam or SmI2...
). Reductive desulfonylation is typically accomplished with active metals or salts (sodium amalgam
Sodium amalgam
Sodium amalgam, commonly denoted Na, is an alloy of mercury and sodium. The term amalgam is used for alloys, intermetallic compounds, and solutions involving mercury as a major component. Sodium amalgam is often used in reactions as strong reducing agents with better handling properties compared...
, aluminium amalgam, magnesium
Magnesium
Magnesium is a chemical element with the symbol Mg, atomic number 12, and common oxidation number +2. It is an alkaline earth metal and the eighth most abundant element in the Earth's crust and ninth in the known universe as a whole...
, samarium(II) iodide
Samarium(II) iodide
Samarium iodide is a green solid composed of samarium and iodine, with a melting point of 520 °C where the samarium atom has a coordination number of seven in a capped octahedral configuration...
), tin hydrides (tributyltin hydride
Tributyltin hydride
Tributyltin hydride is an organotin compound with the formula 3SnH. It is a colorless liquid that is soluble in organic solvents. The compound is used as a source of hydrogen atoms in organic synthesis.-Synthesis and characterization:...
), or transition metal complexes with reducing agents or nucleophiles (PdCl2(dppp)/LiHBEt3, Pd(PPh3)4
Tetrakis(triphenylphosphine)palladium(0)
Tetrakispalladium is the chemical compound Pd[P3]4, often abbreviated Pd4, or even PdP4. It is a bright yellow crystalline solid that becomes brown upon decomposition in air.-Structure and properties:...
/LiHBEt3, Pd(PPh3)4
Tetrakis(triphenylphosphine)palladium(0)
Tetrakispalladium is the chemical compound Pd[P3]4, often abbreviated Pd4, or even PdP4. It is a bright yellow crystalline solid that becomes brown upon decomposition in air.-Structure and properties:...
/NaHC(CO2Et)2). Alkyl, alkenyl, and allylic sulfones may be reduced using one or more of these methods.
(1)
Reductive desulfonylation
Reductive desulfonylation reactions lead to the replacement of a carbon-sulfur bond in the sulfonyl group with a carbon-hydrogen bond. Because the sulfonyl group is by definition attached to two carbons, however, reduction to two sets of products is possible. Mechanistic studies of reductions employing metal amalgams as the reducing agent suggest that upon electron transfer to the sulfone, fragementation to a sulfinate anion and the more stable organic radical occurs. Immediate reduction of the radical and protonation then occur to afford the sulfur-free product derived from the more stable radical. Thus, S-alkyl bonds are cleaved in preference to S-aryl or S-alkenyl bonds.(2)
Samarium(II) iodide may be used to reductively cleave α-keto sulfones; in the presence of hexamethylphosphoramide (HMPA), SmI2 is able to effect reductive elimination of α-functionalized sulfones (see equation (11) below).
(3)
Tin hydrides reduce α-keto and allylic sulfones. The mechanisms of these processes involve the addition of a tin-centered radical to the substrate followed by elimination of a sulfinyl radical, which abstracts a hydrogen from a molecule of tin hydride to propagate the radical chain. Protonation of the organotin intermediates thus formed (by sulfinic acid generated in situ) leads to reduced products. Addition of a stoichiometric amount of proton source allows the use of tin hydride in catalytic amounts. Although desulfonylations of allylic sulfones are site selective (providing only products of allylic transposition), they are not stereoselective and afford mixtures of double bond isomers. The mechanism of desulfonylation of α-keto sulfones is similar.
(4)
Transition-metal-mediated reductive desulfonylations rely on the generation of an intermediate π-allyl complex, which undergoes nucleophilic attack by hydride or another nucleophile to afford reduced products. Nucleophilic attack generally occurs at the less substituted position position of the π-allyl moiety, although site selectivity depends strongly on the substrate and reaction conditions. Palladium(0) complexes are the most commonly used precatalysts.
(5)
Reductive elimination
Sulfones with a good leaving group in the β position may undergo reductive elimination under desulfonylation conditions to afford alkenes. This process is a key step of the Julia olefinationJulia olefination
The Julia olefination is the chemical reaction of phenyl sulfones with aldehydes to give alkenes after alcohol functionalization and reductive elimination using sodium amalgam or SmI2...
, which yields alkenes via addition of an α-sulfonyl carbanion to an aldehyde followed by reductive elimination. Sodium amalgam or samarium(II) iodide/HMPA may be used to convert β-sulfonyloxy or β-acyloxy sulfones to the corresponding alkenes. The key mechanistic step of this process is elimination of an anionic or organometallic intermediate to generate the alkene.
(6)
The use of sodium amalgam, which promotes the formation of essentially free alkyl anions, leads to (E) alkenes with extremely high selectivity. Samarium(II) iodide also produces the (E) alkene predominantly, but with lower selectivity.
Scope and limitations
Using the appropriate reagent and conditions, alkyl, alkenyl, allylic, and α-keto sulfones may be reduced in good yield and high stereoselectivity (where applicable). Appropriate conditions for the reduction of these classes of sulfones are discussed below.Alkyl sulfones may be reduced with sodium or lithium in liquid ammonia; however, the strongly basic conditions of these dissolving metal reductions represent a significant disadvantage. In alcoholic solvents, magnesium metal and a catalytic amount of mercury(II) chloride may be used. A wide variety of functional groups are unaffected by these conditions, including many that are transformed by dissolving metal reductions. Reductive desulfonylation with these reagents does not occur in reactions of β-hydroxy sulfones, due to the poor leaving group ability of the hydroxyl group.
(7)
A significant issue associated with the reduction of allylic sulfones is transposition of the allylic double bond, which occurs in varying amounts during reductions by metal amalgams. and tin hydrides Palladium-catalyzed reductive desulfonylations of allylic sulfones do not have this issue, and afford allylic sulfones with high site and stereoselectivity.
(8)
Aluminum amalgam (Al/Hg) may be used for the chemoselective reduction of α-sulfonylated carbonyl groups. Carboxylic acid derivatives, acetals, thioacetals, amines, alcohols, and isolated double bonds are all inert to Al/Hg. Selective desulfonylation may be carried out on β-hydroxy sulfones without reductive elimination.
(9)
Transition metal catalysis is also useful for the stereospecific reduction of alkenyl sulfones. In the presence of an excess of a Grignard reagent, a palladium(II) or nickel(II) catalyst, and a phosphorus or nitrogen ligand, alkenyl sulfones are converted to the corresponding alkenes stereospecifically in good yield. On the other hand, dissolving metal and metal amalgam reductions are not stereoselective in general. Palladium catalysis is generally superior to nickel catalysis, giving higher yields and stereoselectivities.
(10)
Alkyl and alkenyl sulfones with good leaving groups in the β position undergo elimination under reductive conditions to afford alkenes or alkynes. The Julia olefination
Julia olefination
The Julia olefination is the chemical reaction of phenyl sulfones with aldehydes to give alkenes after alcohol functionalization and reductive elimination using sodium amalgam or SmI2...
exploits this process for the synthesis of alkenes from alkyl sulfones and carbonyl compounds. Addition of an α-sulfonyl anion to a carbonyl compound, followed by quenching with an acyl or sulfonyl chloride, leads to a β-acyloxy or -sulfonyloxy sulfone, which undergoes elimination under reductive conditions. Sodium amalgam may be used to accomplish the elimination step; however, the combination of samarium(II) iodide and HMPA is milder than strongly basic sodium amalgam and leads to higher yields in reductive elimination processes.
(11)
Synthetic applications
The modest acidity of carbons adjacent to the sulfonyl group has made sulfones useful for organic synthesis. Upon removal of the sulfonyl group with desulfonylation or reductive elimination, the net result is the formation of a carbon-carbon bond single or double bond between two unfunctionalized carbons, a ubiquitous motif in synthetic targets. In a synthesis of (–)-anthoplalone, Julia olefination was used to establish the (E)-alkene in the target.(12)
Reductive desulfonylation is employed when the establishment of a carbon-carbon single bond is the goal. In a synthesis of (+)-chatancin, alkylation of an α-sulfonyl carbanion followed by desulfonylation established a key allylic carbon-carbon bond.
(13)
Comparison with other methods
As α-cyano carbanions may be used in many of the same contexts as α-sulfonyl anions, reductive decyanation methods offer a viable alternative to reductive desulfonylation. Dissolving metal reductions are most useful for the decyanation of tertiary nitriles (primary and secondary nitriles give the corresponding amines in addition to decyanated products), but potassium is a more general reducing agent that reduces primary, secondary, and tertiary nitriles.(14)
A wide variety of carbonyl olefination methods that are direct alternative to the Julia olefination are known: the Wittig reaction
Wittig reaction
The Wittig reaction is a chemical reaction of an aldehyde or ketone with a triphenyl phosphonium ylide to give an alkene and triphenylphosphine oxide....
, the Horner-Wadsworth-Emmons reaction
Horner-Wadsworth-Emmons reaction
The Horner-Wadsworth-Emmons reaction is the chemical reaction of stabilized phosphonate carbanions with aldehydes to produce predominantly E-alkenes....
, Peterson olefination
Peterson olefination
The Peterson olefination is the chemical reaction of α-silyl carbanions 1 with ketones to form a β-hydroxysilane 2 which eliminates to form alkenes 3.Several reviews have been published....
, and others. The primary advantage of Julia olefination is that the sulfone precursors are sometimes more readily available and easier to purify than the corresponding phosphorus- or silicon-containing compounds. Additionally, a variety of methods to synthesize sulfones exist. Nonetheless, the sometimes limited stereoselectivity (and in particular, the difficulty of accessing (Z)-alkenes) of the Julia reaction can be problematic. Many alternative methods for olefination, including the Peterson reaction, do not have this issue.
(15)
Typical conditions
Methods employing active metals for reductive desulfonylation require the use of a large excess of the reducing agent. All reactions should be carried out in an inert atmosphere under anhydrous conditions. Although many of the more common reducing agents are commercially available, preparation just prior to use is advised, as many of the reducing agents described below decompose over time.Reductions employing an excess of alkali metals in ammonia or amines are extremely rapid and should be quenched after a minute or so of reaction time—longer reaction times promote side reactions. When lithium or sodium in ammonia is used, the persistance of a deep blue color in the solution (due to solvated electrons) is a sign that the reaction is complete. Reductions employing alkali metal arene radical anion complexes similarly require a large excess of the reducing agent, very low temperatures and short reaction times. Reductive desulfonylations and reductive eliminations employing alkali or alkaline earth metals in ammonia present a high fire hazard and should be conducted in a properly functioning chemical fume hood away from flammable solvents. Ammonia is a corrosive gas with a pungent odor. Alkali metals react violently with water or even moist air to generate hydrogen, which can then be ignited by the heat of the reaction.
In pure alcoholic solvents, magnesium metal is the most commonly employed reducing agent. The required reaction temperature varies substantially across different substrates, although room temperature is usually preferred.
Sodium amalgam containing 2–6% sodium (prepared by the treatment of ribbons of sodium metal with mercury(0)) is the most commonly employed reagent for the reductive desulfonylation and reductive elimination processes. Disodium hydrogen phosphate is used to buffer the solution at a pH of 7–8, meaning that base-labile functionality is stable under the reduction conditions. Reductions employing aluminum amalgam (prepared by immersion of aluminum foil into a 2% aqueous solution of HgCl2) often require higher temperatures than the corresponding sodium amalgam reactions. Mercury and mercury compounds are poisonous and teratogenic, and should be used only in a properly functioning chemical fume hood. Mercury cannot be destroyed but it can be removed from aqueous solutions by using ion-exchange resins or by amalgamation with iron.
Samarium(II) iodide may be easily prepared by the reduction of samarium metal with organic dihalides in THF. It is most often used directly as a solution in THF. Hexamethylphosphoramide increases the reducing power of SmI2 substantially.
Tin halide reductions are almost always accomplished with tributyltin hydride and azobis(isobutyronitrile) (AIBN) as a radical initiator. Tin hydrides are toxic irritants and should be handled with care in a fume hood.
Among transition-metal-mediated reactions, the use of [PdCl2(dppp)] and superhydride (LiHBEt3) at low temperatures is preferred for reductions of allylic sulfones. Lithium triethylborohydride is supplied as a 1 M solution in tetrahydrofuran
Tetrahydrofuran
Tetrahydrofuran is a colorless, water-miscible organic liquid with low viscosity at standard temperature and pressure. This heterocyclic compound has the chemical formula 4O. As one of the most polar ethers with a wide liquid range, it is a useful solvent. Its main use, however, is as a precursor...
, is corrosive and flammable, and should be stored under an inert atmosphere in a cool, dry place. Vinylic sulfones may be reduced with palladium(II) catalysts such as [Pd(acac)2] and Grignard reagents in tetrahydrofuran at room temperature. Tetrahydrofuran solutions of n-BuMgCl are highly flammable, sensitive to moisture, and cause burns. Homogeneous organonickel complexes, used most often in excess in THF at room temperature, are used to reduce alkyl sulfones.
Example procedure
(16)Argon was bubbled into a solution of (S, E)-methyl 2-benzamido-4-fluoro-2-methyl-4-(phenylsulfonyl)but-3-enoate (41 mg, 0.10 mmol) in benzene (1 mL) for 2 minutes. Tributyltin hydride (64 mg, 0.20 mmol) and AIBN (2.0 mg, 0.01 mmol) were then added under an argon atmosphere. The reaction mixture was heated under reflux for 24 hours, concentrated, and the residue was chromatographed (hexane to 90:10 hexane/EtOAc) to give the tri-n-butylstannyl vinylalaninate derivative (45 mg, 80%): IR (film) 1741, 1525 cm–1; 1H NMR (500 MHz, CDCl3) δ 0.85 (m, 9H), 0.99 (m, 6H), 1.30 (m, 6H), 1.50 (m, 6H), 1.81 (s, 3H), 3.78 (s, 3H), 5.38 (d, J = 56.0 Hz, 1H), 7.40 (m, 2H), 7.46 (d, J = 7.0 Hz, 1H), 7.49 (bs, 1H), 7.77 (m, 2H); 13C NMR 125 MHz, (CDCl3) δ 8.7, 10.1, 13.5, 13.6, 24.3, 26.8, 27.0, 27.2, 28.6, 28.7, 28.8, 52.9, 57.9, 58.0, 124.2, 127.0, 127.1, 128.4, 131.4, 134.6, 165.9, 172.2, 173.9, 174.7; 19F NMR (470 MHz, CDCl3) δ –94.99 (d, J = 55.0 Hz). Anal. Calcd for C25H40NO3FSn: C, 55.58; H, 7.46; N, 2.59. Found: C, 54.85; H, 7.34; N, 2.54.
A suspension of the tri-n-butylstannyl vinylalaninate (46.4 mg, 0.1 mmol) in 6 N HCl (2 mL) was refluxed for 17 hours. Following sequential extraction with CH2Cl2 and EtOAc, the aqueous layer was evaporated under vacuum and mild heating (40°) to give the title product salt (13.4 mg, 89%): 1H NMR (500 MHz, D2O) δ 1.73 (s, 3H), 5.21 (dd, J = 44.0, 5.0 Hz, 1H), 6.77 (dd, J = 82.0, 5.0 Hz, 1H); 19F NMR (470 MHz, CDCl3) δ –117.85 (dd, J = 82.0, 43.0 Hz); HRMS–FAB (m/z): [M + H]+ calcd for C5H9FNO2,134.0617; found, 134.0616.