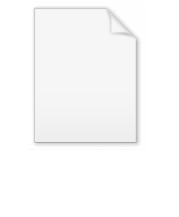
Type II topoisomerase
Encyclopedia
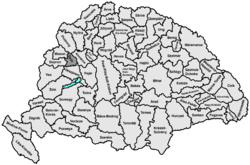
Adenosine triphosphate
Adenosine-5'-triphosphate is a multifunctional nucleoside triphosphate used in cells as a coenzyme. It is often called the "molecular unit of currency" of intracellular energy transfer. ATP transports chemical energy within cells for metabolism...
, unlike type I topoisomerase
Topoisomerase
Topoisomerases are enzymes that regulate the overwinding or underwinding of DNA. The winding problem of DNA arises due to the intertwined nature of its double helical structure. For example, during DNA replication, DNA becomes overwound ahead of a replication fork...
. In this process, these enzymes change the linking number
Linking number
In mathematics, the linking number is a numerical invariant that describes the linking of two closed curves in three-dimensional space. Intuitively, the linking number represents the number of times that each curve winds around the other...
of circular DNA by +/-2.
Function
Once cut, the ends of the DNA are separated, and a second DNA duplex is passed through the break. Following passage, the cut DNA is religated. This reaction allows type II topoisomerases to increase or decrease the linking number of a DNA loop by 2 units, and promotes chromosome disentanglement. Reactions involving the increase in supercoiling require two molecules of ATPAdenosine triphosphate
Adenosine-5'-triphosphate is a multifunctional nucleoside triphosphate used in cells as a coenzyme. It is often called the "molecular unit of currency" of intracellular energy transfer. ATP transports chemical energy within cells for metabolism...
. Janet Lindsley has done much work to examine how the hydrolysis of ATP
Adenosine triphosphate
Adenosine-5'-triphosphate is a multifunctional nucleoside triphosphate used in cells as a coenzyme. It is often called the "molecular unit of currency" of intracellular energy transfer. ATP transports chemical energy within cells for metabolism...
translates to topoisomerase function. For example, DNA gyrase
DNA gyrase
DNA gyrase, often referred to simply as gyrase, is an enzyme that relieves strain while double-stranded DNA is being unwound by helicase. This causes negative supercoiling of the DNA...
, a type II topoisomerase observed in E. coli and most other prokaryote
Prokaryote
The prokaryotes are a group of organisms that lack a cell nucleus , or any other membrane-bound organelles. The organisms that have a cell nucleus are called eukaryotes. Most prokaryotes are unicellular, but a few such as myxobacteria have multicellular stages in their life cycles...
s, introduces negative supercoils and decreases the linking number by 2. Gyrase is also able to remove knots from the bacterial chromosome
Chromosome
A chromosome is an organized structure of DNA and protein found in cells. It is a single piece of coiled DNA containing many genes, regulatory elements and other nucleotide sequences. Chromosomes also contain DNA-bound proteins, which serve to package the DNA and control its functions.Chromosomes...
. Along with gyrase, most prokaryotes also contain a second type IIA topoisomerase, termed topoisomerase IV. Gyrase and topo IV differ by their C-terminal domains, which is believed to dictate substrate specificity and functionality for these two enzymes. Footprinting indicates that gyrase, which forms a 140-base-pair footprint and wraps DNA, allowing it to introduce negative supercoils, while topo IV, which forms a 28-base-pair footprint, does not wrap DNA.
Eukaryotic type II topoisomerase cannot introduce supercoils; it can only relax them.
The role of type IIB topoisomerases is less understood. Unlike type II topoisomerases, it cannot simplify DNA topology (see below), but it shares several structural features with type IIA topoisomerases.
Topology simplification
Type IIA topoisomerases are essential in the separation of entangled daughter strands during replication. This function is believed to be performed by topo II in eukaryotes and by topo IV in prokaryotes. Failure to separate these strands leads to cell death. Type IIA topoisomerases have the special ability to relax DNA to a state below that of thermodynamic equilibrium, a feature unlike type IA, IB, and IIB topoisomerases. This ability, known as topology simplification, was first identified by Rybenkov et al. (Science 1997). The hydrolysis of ATP drives this simplification, but a clear molecular mechanism for this simplification is still lacking. Several models to explain this phenomenon have been proposed, including two models that rely on the ability of type IIA topoisomerases to recognize bent DNA duplexes (Vologodskiy, Proceedings of the National Academy of Science 1999). Biochemistry, electron microscopy, and recent structures of topo II bound to DNA reveal that type IIA topoisomerases bind at the apices of DNA, supporting this model.Classification
There are two subclasses of type II topoisomerases, type IIA and IIB.- Type IIA topoisomerases include the enzymes DNA gyrase, eukaryotic topoisomerase II (topo II), and bacterial topoisomerase IVTopoisomerase IVTopoisomerase IV is one of two type-II topoisomerases in bacteria, the other being DNA gyrase. Like gyrase, topoisomerase IV is able to pass one double-strand of DNA through another double-strand of DNA, thereby changing the linking number of DNA by two in each enzymatic...
(topo IV). These enzymes span all domains of life and are essential for function. - Type IIB topoisomerases are structurally and biochemically distinct, and comprise a single family member, topoisomerase VI (topo VI). Type IIB topoisomerases are found in archaea and some higher plants.
Some organisms have two isoforms of topoisomerase II: alpha and beta. In cancers, the topoisomerase II-alpha is highly expressed in highly proliferating cells. In certain cancers, such as peripheral nerve sheath tumors, high expression of its encoded protein is also associated to poor patient survival.
The two classes of topoisomerases possess a similar strand passage mechanism and domain structure (see below), however they also have several important differences. Type IIA topoisomerases form double-stranded breaks with four-base pair overhangs, while type IIB topoisomerases form double-stranded breaks with two base overhangs (Buhler, Lebbink, Bocs, Ladenstein, and Forterre, Journal of Biological Chemistry 2001). In addition, type IIA topoisomerases are able to simplify DNA topology (Rybenkov Science 1997), while type IIB topoisomerases do not (Corbett Journal of Molecular Biology, 2006).
Structure of type IIA topoisomerases
Type IIA topoisomerases consist of several key motifs:an N-terminal GHKL ATPase domain (for Gyrase, Hsp, Kinase and MutL),
a Toprim domain (sometimes called a Rossmann fold), which exists in both Type II topoisomerases, type IA topoisomerases, and bacterial primase (DnaG), a central DNA-binding core (which structurally forms a heart-shape structure), and a variable C-terminal domain.
Eukaryotic type II topoisomerases are homodimers (A2), while prokaryotic type IIs are heterodimers (A2B2). Prokaryotes have the ATPase domain and the Toprim fold on one polypeptide, while the DNA cleavage core and the CTD lies on a second polypeptide. For gyrase, the first polypeptide is called GyrB and the second polypeptide is called GyrA. For topo IV, the first polypeptide is called ParE and the second polypeptide is called ParC.
The structure of the N-terminal ATPase domain of gyrase (Wigley, Davies, Dodson, Maxwell, and Dodson, Nature 1991) and yeast topo II (Classen and Berger, Proceedings of the National Academy of Science, 2003, PDB ID=1PVG) have been solved in complex with AMPPNP (an ATP analogue), showing that two ATPase domains dimerize to form a closed conformation. For gyrase, the structure has a substantial hole in the middle, which is presumed to accommodate the T-segment.
Linking the ATPase domain to the Toprim fold is a helical element known as the transducer domain. This domain is thought to communicate the nucleotide state of the ATPase domain to the rest of the protein. Modifications to this domain affects topoisomerase activity, and structural work done by the Verdine group shows that the ATP state affects the orientation of the transducer domain (Journal of Biological Chemistry, 2006).
The central core of the protein contains a Toprim fold and a DNA-binding core that contains a Winged Helix domain (WHD), often referred to as a CAP domain, since it was first identified to resemble the WHD of Catabolite Activator Protein. The catalytic tyrosine lies on this WHD. The Toprim fold is a Rossmann fold that contains three invariant acidic residues that coordinate magnesium ions involved in DNA cleavage and DNA religation (Avarind, Leipe, Konin, Nucleic Acids Research 1998). The structure of the Toprim fold and DNA-binding core of yeast topo II was first solved by Berger and Wang (Nature 1996, PDB ID = 1BGW) and the first gyrase DNA-binding core was solved by Morais Cabral et al. (Nature 1997, PDB ID = 1AB4). The structure solved by Berger revealed important insights into the function of the enzyme. The DNA-binding core consists of the WHD, which leads to a tower domain. A coiled-coil region leads to a C-terminal domain that forms the main dimer interface for this crystal state (often termed the C-gate). It is interesting to note that, while the original topo II structure shows a situation where the WHDs are separated by a large distance, the structure of gyrase shows a closed conformation, where the WHD close.
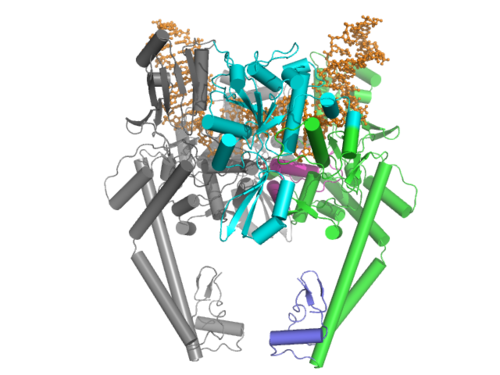
More recently, several structures of the DNA-bound structure have been solved in an attempt to understand both the chemical mechanism for DNA cleavage and the structural basis for inhibition of topoisomerase by antibacterial poisons.
The C-terminal region of the prokayrotic topoisomerases have been solved in multiple species. The first structure of a C-terminal domain of gyrase was solved by Corbett et al. (Proceedings of the National Academy of Science, 2004, PDB ID = 1SUU) and the C-terminal domain of topo IV was solved by Corbett et al. (Journal of Molecular Biology, 2006, PDB ID = 1zvt and 1zvu). The structures formed a novel beta barrel, which bends DNA by wrapping the nucleic acid around itself. The bending of DNA by gyrase has been proposed as a key mechanism in the ability of gyrase to introduce negative supercoils into the DNA. This is consistent with footprinting data that shows that gyrase has a 140-base-pair footprint. It is interesting to note that both gyrase and topo IV CTDs bend DNA, but only gyrase introduces negative supercoils.
Unlike the function of the C-terminal domain of prokaryotic topoisomerases, the function of the C-terminal region of eukaryotic topoisomerase II is still not clear. Studies have suggested that this region is regulated by phosphorylation and this modulates topoisomerase activity, however more research needs to be done to investigate this.
Structures of type IIB topoisomerases
The organization of type IIB topoisomerases are similar to that of type IIAs, except that all type IIBs have two genes and form heterodimers. One gene, termed topo VI-B (since it resembles gyrB), contains the ATPase domain, a H2TH domainH2TH domain
In molecular biology, the H2TH domain is a DNA-binding domain found in DNA glycosylase/AP lyase enzymes, which are involved in base excision repair of DNA damaged by oxidation or by mutagenic agents. Most damage to bases in DNA is repaired by the base excision repair pathway...
, and the transducer domain. The second gene, termed topo VI-A, contains the WHD and the Toprim domain.
The ATPase domain of topo VI B was solved in multiple nucleotide states (Corbett and Berger, EMBO J 2003). It closely resembles that of the GHKL domain of topo II and MutL and shows that the nucleotide state (ADP versus ATP) effects the orientation of the transducer domain (pdb ID= 1MU5 and 1MX0).
The structure of topo VI-A was solved by Bergerat et al. (Nature 1997), showing that the HTH and Toprim fold had a novel conformation compared with that of topo IIA.
A recent structure of the topo VI A/B complex was solved, showing an open and closed conformation, two states that are predicted in the two-gate mechanism (see below). These structures, of which one is an X-ray crystal structure and the other is a Small-Angle X-ray Scattering (SAXS) reconstruction, show that the ATPase domain can be either open or closed (Corbett, Benedetti, Berger Nature Structure Molecular Biology, 2007, PDB ID = 2Q2E).
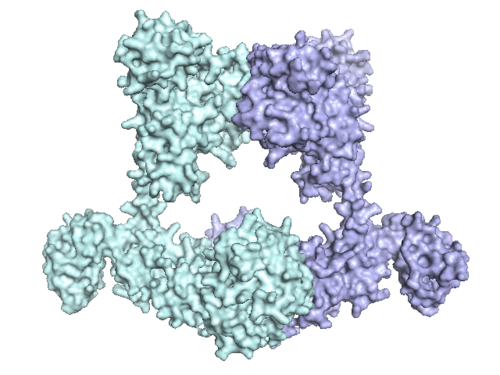
Strand Passage Mechanism of type II topoisomerases
Type IIA topoisomerase operates through a "two-gate" mechanism (though this is a historical notation), a mechanism supported by biochemistry (Roca and Wang) as well as by structural work (Berger and Wang) (see above).A strand of DNA, called the Gate, or G-segment is bound by a central DNA-binding gate (DNA-gate). A second strand of DNA, called the Transport, or T-segment, is captured by the dimerization of the N-terminal ATPase domain (the ATPase-gate) when two molecules of ATP bind. Hydrolysis of ATP and release of an inorganic phosphate leads to the cleavage of the G-segment, as the catalytic tyrosines form a covalent phosphotyrosine bond with the 5' end of the DNA. This creates a four-base overhang and a double-stranded break in the G-segment. As the DNA-binding gate separates, the T-segment is transferred through the G-segment. The G-segment is sealed, leading to the C-terminal gate (or C-gate) to open, allowing for the release of the T-segment. Release of product ADP leads to a reset of the system, and allows a second T-segment to be captured.
Type IIB topoisomerases operate through a similar fashion, except that the protein forms a two-base overhang in the G-segment and that the C-terminal gate is completely missing.
DNA cleavage mechanism of type IIA topoisomerases
In the strand passage mechanism, the cleavage of DNA is key to allow the T-segment to transfer through the G-segment. The mechanism of DNA cleavage by type IIA topoisomerases has recently been the focus of many biochemical and structural biology studies.Catenation
CatenationCatenation
Catenation is the ability of a chemical element to form a long chain-like structure via a series of covalent bonds. Catenation occurs most readily in carbon, which forms covalent bonds with other carbon atoms. Catenation is the reason for the presence of a large number of organic compounds in nature...
is the process by which two circular DNA strands are linked together like chain links. This occurs after DNA replication, where two single strands are catenated and can still replicate but cannot separate into the two daughter cells. As Type II topoisomerses break a double strand, they can fix this state (Type I topoisomerases could do this only if there was already a single strand nick), and the correct chromosome number can remain in daughter cells. Linear DNA in eukaryotes is so long they can be thought of as being without ends; Type II topoisomerases are needed for the same reason.
Inhibition
Small molecules that target type II topoisomerase are divided into two classes: inhibitors and poisons.- Inhibitors of type II topoisomerase include HU-331HU-331HU-331 is a quinone anticarcinogenic drug synthesized from cannabidiol, a cannabinoid in the Cannabis sativa plant. It showed a great efficacy against oncogenic human cells. HU-331 does not cause arrest in cell cycle, cell apoptosis or caspase activation...
, ICRF-187, ICRF-193, and mitindomide. These molecules work by inhibiting the ATPase activity by acting as a non-competitive inhibitor of ATP. This has been shown through structural studies (Classen et al. Proceedings of the National Academy of Science, 2005) and biochemical studies performed by the Lindsley group.
- Poisons of type II topoisomerases include etoposideEtoposideEtoposide phosphate is an anti-cancer agent. It is known in the laboratory as a topoisomerase poison. Etoposide is often incorrectly referred to as a topoisomerase inhibitor in order to avoid using the term "poison" in a clinical setting...
, NovobiocinNovobiocinNovobiocin, also known as albamycin or cathomycin, is an aminocoumarin antibiotic that is produced by the actinomycete Streptomyces niveus, which has recently been identified as a subjective synonym for S. spheroides a member of the order Actinobacteria . Other aminocoumarin antibiotics include...
, QuinoloneQuinoloneThe quinolones are a family of synthetic broad-spectrum antibiotics. The term quinolone refers to potent synthetic chemotherapeutic antibacterials....
(including CiprofloxacinCiprofloxacinCiprofloxacin is a synthetic chemotherapeutic antibiotic of the fluoroquinolone drug class.It is a second-generation fluoroquinolone antibacterial. It kills bacteria by interfering with the enzymes that cause DNA to rewind after being copied, which stops synthesis of DNA and of...
), and teniposideTeniposideTeniposide is a chemotherapeutic medication mainly used in the treatment of childhood acute lymphocytic leukemia . It is in a class of drugs known as podophyllotoxin derivatives and slows the growth of cancer cells in the body....
. These small molecules target the DNA-protein complex. Some of these molecules lead to increased cleavage, whereas others, such as etoposide, inhibit religation.
The experimental anti-tumor drug m-AMSA (4'-(9'-acridinylamino)methanesulfon-m-anisidide) also inhibits type 2 topoisomerase.
Topoisomerase poisons have been extensively used as both anti-cancer and anti-bacterial therapies. It is interesting to note that, while antibacterial compounds such as ciprofloxacin target bacterial gyrase, they fail to inhibit eukaryotic type IIA topoisomerases. In addition, drug-resistance bacteria often have a point mutation in gyrase (Serine79Alanine in E. coli), that renders quinolones ineffective. Recent structural studies have led to the discovery of a compound that no longer relies on this residue and, therefore, has efficacy against drug-resistant bacteria.