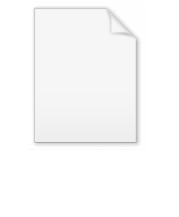
Arieh Warshel
Encyclopedia
Arieh Warshel is a Distinguished Professor of Chemistry and Biochemistry at the University of Southern California
. He is known for his work on computational biochemistry and biophysics, and, in particular, for having pioneered computer simulations of the function of biological systems, and for developing what is now known as Computational Enzymology.
Arieh Warshel was born in Kibbutz Sde-Nahum in Israel in 1940. After serving in the Israeli Army (final rank Captain), he attended the Technion in Haifa, where he received his BSc degree in Chemistry, Summa Cum Laude, in 1966. He subsequently earned both MSc and PhD degrees in Chemical Physics (1967 and 1969 respectively) from the Weizmann Institute of Science, together with Shneior Lifson. After his PhD, he did postdoctoral work at Harvard University. From 1972 to 1976, he was at the Weizmann Institute and at the MRC Laboratory for Molecular Biology in Cambridge, England. In 1976, he joined the faculty of the Department of Chemistry at USC, where he is a Distinguished Professor of Chemistry and Biochemistry.
Warshel has been elected a member of the United States National Academy of Sciences
, a fellow of the Royal Society of Chemistry
(FRSC), and a fellow of the Biophysical Society (2000). He was additionally awarded the Technion Award for best third-year student in Chemistry (1965), the Mifal-Hapays Prize (1969), the Alfred P. Sloan Fellowship (1978-1980), the USC Associated Award for Creativity in Research (1981), the 1993 Annual Award of the International Society of Quantum Biology and Pharmacology, the Tolman Medal (2003), and the 2006 President’s Award for Computational Biology from the ISQBP.
Warshel introduced some of the most crucial approaches in modeling protein function. He also pioneered (and co-pioneered) key studies and directions that turned out eventually to be essential for quantifying protein function. These include:
In 1976, Warshel and Levitt pioneered calculations that considered an entire enzyme-substrate complex in solution, and evaluated the catalytic effect of this system . This work introduced the hybrid quantum mechanical / molecular mechanical (QM/MM) approach, which has since become a major scientific discipline. Warshel’s subsequent studies paved the way for the quantitative modeling of enzyme reactions. He has pioneered simulations of the dynamics of enzymatic reactions, and introduced the powerful free energy perturbation method for the modeling of enzymatic reactions. Warshel proposed and quantitatively established that enzyme catalysis is due to the fact that the active site dipoles are already preoriented towards the charge distribution of the transition state . His key advances in this direction include:
The first use of MD in studying a biological process was presented by Warshel in 1976 . His simulations of the primary event in the vision process correctly predicted the photoisomerization time of rhodopsin. This prediction has been subsequently experimentally confirmed . Warshel also used the same semiclassical approach in pioneering simulations of the primary electron transfer in photosynthesis . Warshel’s 1976 simulations of photochemical processes also discovered a very large crossing probability, that reflected what is now known as the effect of conical intersections. This feature has been now confirmed in many photobiological reactions (e.g., . His key advances in this direction include:
Warshel’s works paved the way for quantitative studies of electrostatic energies in proteins. He and his coworkers provided the first treatments that considered the entire contributions to the electrostatic free energies of proteins . He also introduced the first simplified microscopic treatment of the energy of charges in solvated proteins and the first free energy perturbation study of a charge in a protein . His models opened the way to realistic calculations of pKas, redox potentials and absolute binding free energies in proteins. His key advances in this direction include:
Warshel pioneered the simulation of electron transfer processes in the condensed phase . He developed microscopic free energy functions and introduced the potential energy gap as a generalized reaction coordinate . His approach provides the microscopic equivalent of Marcus’ theory. He also pioneered the dispersed polaron model, which paved the way for simulations of quantum tunneling in biological systems . Warshel and coworkers presented the first simulation of electron-transfer dynamics in bacterial reaction centers , and correctly predicted that a three-step mechanism is the actual mechanism . His key advances in this direction include:
Warshel also pioneered computational methods for the microscopic studies of chemical processes in polar solvents . His contributions include the introduction of QM/MM methods to studies of reactions in solution . Another major accomplishment was the development of the EVB method and the introduction of free energy perturbation calculations of activation free energies of reactions in solution . These methods led to the first microscopic calculations of the dynamics and energetics of charge transfer reactions in polar solvents and in enzymes [ref]. The EVB approach has become widely used in many applications, including in enzyme design . His key advances in this direction include:
Laying the Basis for Modern Force Fields
University of Southern California
The University of Southern California is a private, not-for-profit, nonsectarian, research university located in Los Angeles, California, United States. USC was founded in 1880, making it California's oldest private research university...
. He is known for his work on computational biochemistry and biophysics, and, in particular, for having pioneered computer simulations of the function of biological systems, and for developing what is now known as Computational Enzymology.
Arieh Warshel was born in Kibbutz Sde-Nahum in Israel in 1940. After serving in the Israeli Army (final rank Captain), he attended the Technion in Haifa, where he received his BSc degree in Chemistry, Summa Cum Laude, in 1966. He subsequently earned both MSc and PhD degrees in Chemical Physics (1967 and 1969 respectively) from the Weizmann Institute of Science, together with Shneior Lifson. After his PhD, he did postdoctoral work at Harvard University. From 1972 to 1976, he was at the Weizmann Institute and at the MRC Laboratory for Molecular Biology in Cambridge, England. In 1976, he joined the faculty of the Department of Chemistry at USC, where he is a Distinguished Professor of Chemistry and Biochemistry.
Warshel has been elected a member of the United States National Academy of Sciences
United States National Academy of Sciences
The National Academy of Sciences is a corporation in the United States whose members serve pro bono as "advisers to the nation on science, engineering, and medicine." As a national academy, new members of the organization are elected annually by current members, based on their distinguished and...
, a fellow of the Royal Society of Chemistry
Royal Society of Chemistry
The Royal Society of Chemistry is a learned society in the United Kingdom with the goal of "advancing the chemical sciences." It was formed in 1980 from the merger of the Chemical Society, the Royal Institute of Chemistry, the Faraday Society and the Society for Analytical Chemistry with a new...
(FRSC), and a fellow of the Biophysical Society (2000). He was additionally awarded the Technion Award for best third-year student in Chemistry (1965), the Mifal-Hapays Prize (1969), the Alfred P. Sloan Fellowship (1978-1980), the USC Associated Award for Creativity in Research (1981), the 1993 Annual Award of the International Society of Quantum Biology and Pharmacology, the Tolman Medal (2003), and the 2006 President’s Award for Computational Biology from the ISQBP.
Research Achievements
Warshel has made major contributions in introducing computational methods for studying structure-function correlation in biological molecules. He pioneered (and co-pioneered) simulation software, as well as key methods and concepts for microscopic studies of the functional properties of biological molecules, including the Cartesian-based force field program CFF , the QM/MM method for simulating enzymatic reactions, , molecular dynamics simulations of biological molecules , microscopic electrostatic models for proteins , free energy perturbations in proteins , and other key advances. His contributions include the following:Laying the Foundation for Many Modern Computer Simulations of Protein Function
Warshel introduced some of the most crucial approaches in modeling protein function. He also pioneered (and co-pioneered) key studies and directions that turned out eventually to be essential for quantifying protein function. These include:
Co-introducing (1976) the combined quantum mechanical / molecular mechanical (QM/MM) approach, which has become the basis of the field of computational enzymology . Performing (1976) the first molecular dynamics (MD) simulation of a biological process . Co-introducing the simplified protein-folding model (1975) . Introducing (1981) computer-based microscopic thermodynamic cycles in biology (11). Introducing (1981) consistent calculations of spectral shifts, as well as consistent microscopic calculations of pKas in proteins . Performing (1984) the first free-energy perturbation calculations in proteins . Introducing (1986) consistent structure-based calculations of the redox potentials of proteins . Introducing (1991) simulations of quantum-mechanical tunneling in enzymatic reactions and in solution . Introducing (1992) microscopic linear response approximation (LRA) calculations of absolute binding free energies . Introducing (1999) simulations of time-dependent proton transport in proteins .
Quantifying the Molecular Basis for Enzyme Catalysis
In 1976, Warshel and Levitt pioneered calculations that considered an entire enzyme-substrate complex in solution, and evaluated the catalytic effect of this system . This work introduced the hybrid quantum mechanical / molecular mechanical (QM/MM) approach, which has since become a major scientific discipline. Warshel’s subsequent studies paved the way for the quantitative modeling of enzyme reactions. He has pioneered simulations of the dynamics of enzymatic reactions, and introduced the powerful free energy perturbation method for the modeling of enzymatic reactions. Warshel proposed and quantitatively established that enzyme catalysis is due to the fact that the active site dipoles are already preoriented towards the charge distribution of the transition state . His key advances in this direction include:
Introducing (1976) the combined quantum mechanical / molecular mechanical (QM/MM) approach, which has since become the basis of the field of Computational Enzymology . Proposing (1978) and demonstrating that polar preorganization is the key factor in enzyme catalysis, and rationalizing the inverted catalysis/stability relationship . Pioneering free energy perturbation (1984) calculations of enzymatic reactions, which were subsequently extended to the reactivity of many key enzyme families . Performing (1984) the first simulation of dynamical contributions to enzyme catalysis. Quantifying (2000) entropic contributions to enzyme catalysis . Systematically simulating (1980-2011 ) almost all of the available catalytic proposals, and demonstrating that most enzymes catalyze their reactions by electrostatic effects .
Pioneering MD Simulations of Biological Functions
The first use of MD in studying a biological process was presented by Warshel in 1976 . His simulations of the primary event in the vision process correctly predicted the photoisomerization time of rhodopsin. This prediction has been subsequently experimentally confirmed . Warshel also used the same semiclassical approach in pioneering simulations of the primary electron transfer in photosynthesis . Warshel’s 1976 simulations of photochemical processes also discovered a very large crossing probability, that reflected what is now known as the effect of conical intersections. This feature has been now confirmed in many photobiological reactions (e.g., . His key advances in this direction include:
Simulating (1976) the first step in the vision process, and predicting that this step occurs in about 100fs, while finding that this step involves an enormous crossing probability . The predicted time has been since confirmed experimentally and other features of the calculations, like the large crossing probability (what is now known as a “conical intersection”) as well as the partially concerted isomerization path were eventually confirmed by ab initio QM/MM simulations . Demonstrating (1984) that productive trajectories in enzymatic reactions take about 1ps . Simulating (1985) the autocorrelation time that controls the protein response in charge transport processes [ref]. This prediction was eventually also experimentally confirmed (1988 (Find Experimnts).
Laying the Basis for Microscopic Electrostatic Calculations of Proteins
Warshel’s works paved the way for quantitative studies of electrostatic energies in proteins. He and his coworkers provided the first treatments that considered the entire contributions to the electrostatic free energies of proteins . He also introduced the first simplified microscopic treatment of the energy of charges in solvated proteins and the first free energy perturbation study of a charge in a protein . His models opened the way to realistic calculations of pKas, redox potentials and absolute binding free energies in proteins. His key advances in this direction include:
Introducting (1976) consistent microscopic models of solvated proteins . Establishing (1978) the key role of the polarity of protein active sites . Establishing (1984) the desolvation penalty and the crucial compensation effects of the protein permanent dipoles, and quantifying the energetics of ion pairs in proteins . Introducing (1986) free energy perturbation and LRA calculations (1992) of electrostatic energies in proteins . Performing (1988) the first calculations of the dielectric relaxation of proteins . Introducing (1978) spherical boundary conditions and long-range spherical treatments (1989) . Performing (1991) the first calculations of the dielectric constant of protein active sites (rather than the entire protein) and other selected regions
Introducing Simulations of Electron Transfer Processes in Solution and in Proteins
Warshel pioneered the simulation of electron transfer processes in the condensed phase . He developed microscopic free energy functions and introduced the potential energy gap as a generalized reaction coordinate . His approach provides the microscopic equivalent of Marcus’ theory. He also pioneered the dispersed polaron model, which paved the way for simulations of quantum tunneling in biological systems . Warshel and coworkers presented the first simulation of electron-transfer dynamics in bacterial reaction centers , and correctly predicted that a three-step mechanism is the actual mechanism . His key advances in this direction include:
Introducing (1982) microscopic simulations of electron transfer in solution . Pioneering (1986,1989) the microscopic evaluation of the Marcus free energy functions (and reorganization energies), as well as the simulation of nuclear tunneling effects associated with protein modes . Introducing (1989, 1991) the use of MD simulations (dispersed polaron / spin-boson) to study the effect of protein quantized nuclear motions in electron and proton transfer reactions . Simulating (1988) the primary event in photosynthesis, and predicting a direct hopping mechanism (which was later experimentally confirmed)
Pioneering Simulations of Chemical Reactions in Solution
Warshel also pioneered computational methods for the microscopic studies of chemical processes in polar solvents . His contributions include the introduction of QM/MM methods to studies of reactions in solution . Another major accomplishment was the development of the EVB method and the introduction of free energy perturbation calculations of activation free energies of reactions in solution . These methods led to the first microscopic calculations of the dynamics and energetics of charge transfer reactions in polar solvents and in enzymes [ref]. The EVB approach has become widely used in many applications, including in enzyme design . His key advances in this direction include:
Introducing (1978) calculations of solvent effects on chemical reactions, using realistic yet simplified solvent models . Performing (1982) the first MD simulations of proton transfer reactions in solution . Introducing (1982) the energy gap as the reaction coordinate for studies of reactions in solution and in proteins . Introducing (1980, 1988) the EVB as a general tool for simulating condensed-phase reactions) . Introducing (1986) MD simulations of quantum mechanical nuclear tunneling in electron transfer reactions .
Laying the Basis for Modern Force Fields
In 1967, Warshel realized the great advantages of programming molecular force fields using a Cartesian coordinate representation rather than in internal coordinates as was the norm at the time. His approach enormously simplified the evaluation of analytic derivatives, allowing one to generate large molecules and evaluate the normal modes of large molecules. This was a key factor in the development of the Consistent Force Field (CFF) computer program (in cooperation with M. Levitt and S. Lifson). The CFF program has provided the basis for many features of most of the current macromolecular modeling programs (e.g. CHARMM, AMBER, BIOGRAPH, DISCOVER and GROMOS). He also introduced polarizable force fields and the consistent reactive force field (namely the EVB). His key advances in this direction include:
Introducing (1968) the crucial idea of using Cartesian coordinates in general force field programs . Introducing (1968) vibrational calculations and consistent force field parameterization in molecular mechanics . Co-developing (1969) the program that is the basis of all current molecular simulation programs (1969) [ref]. Pioneering (1976) polarizable force fields . Pioneering (1980) the Empirical Valence Bond .
External links
In 1967, Warshel realized the great advantages of programming molecular force fields using a Cartesian coordinate representation rather than in internal coordinates as was the norm at the time. His approach enormously simplified the evaluation of analytic derivatives, allowing one to generate large molecules and evaluate the normal modes of large molecules. This was a key factor in the development of the Consistent Force Field (CFF) computer program (in cooperation with M. Levitt and S. Lifson). The CFF program has provided the basis for many features of most of the current macromolecular modeling programs (e.g. CHARMM, AMBER, BIOGRAPH, DISCOVER and GROMOS). He also introduced polarizable force fields and the consistent reactive force field (namely the EVB). His key advances in this direction include:
Introducing (1968) the crucial idea of using Cartesian coordinates in general force field programs . Introducing (1968) vibrational calculations and consistent force field parameterization in molecular mechanics . Co-developing (1969) the program that is the basis of all current molecular simulation programs (1969) [ref]. Pioneering (1976) polarizable force fields . Pioneering (1980) the Empirical Valence Bond .